Application of seaweed polysaccharide in bone tissue regeneration
- 1School of Pharmacy, Changchun University of Chinese Medicine, Changchun, Jilin, China
- 2Northeast Asia Research Institute of Traditional Chinese Medicine, Changchun University of Chinese Medicine, Changchun, China
Regeneration is a complex process influenced by many independent or combined factors, including inflammation, proliferation, and tissue remodeling. The ocean, the most extensive resource on Earth, is rich in Seaweed. With increasing research in recent years, researchers have discovered that seaweed polysaccharides have various pharmacological effects, including a particular efficacy in promoting bone tissue regeneration. However, the application of this material in the field of bone tissue engineering is very limited. However, there are few studies on the polysaccharide at home and abroad, and little is known about its potential application value in bone repair. In addition, the bioavailability of the seaweed polysaccharide is also low, and there are still many problems to be solved. For example, the ease of solubility of fucoidan in water is a key issue that restricts its practical application. In this review, we summarize the applications and mechanisms of seaweed polysaccharides in bone healing. We also propose to combine seaweed polysaccharides with novel technologies through different types of preparations, hydrogels, scaffolds, and 3D printing to improve their use in tissue healing and regeneration.
1 Introduction
Bone tissue is a highly dynamic, complex vascularized tissue that undergoes continuous remodeling throughout life (Hoseinpour and Shariatinia, 2021). While bone tissue has a degree of self-healing ability, its ability to repair itself is limited when faced with severe injuries, such as trauma, infection, tumor removal, and joint revision (McKinley et al., 2023). These diseases are beyond the ability of humans to heal themselves, making it difficult to recover through autologous bone tissue regeneration alone. The healing time is long, and patients will suffer great pain. Bone tissue damage often leads to severe disability (Alazzam et al., 2023). Therefore, bone tissue regeneration is becoming a hot research topic, and it is vital to explore promising treatment strategies (Fu et al., 2022). Consequently, a new treatment is needed to promote bone tissue regeneration.
As the largest part of the Earth, the ocean is rich in resources containing different types of plants and animals, including seaweed. As a simple marine plant, seaweed organisms consist mainly of structural components such as extracellular matrix, cell wall, and cell plasma (Giuliani, 2019). Primary and secondary metabolites in seaweed organisms include large molecular weight biomass components (e.g., seaweed polysaccharides, proteins, and brown seaweed starch) and medium, small, and trace components, such as fucoidan polyphenols, rock seaweed polysaccharides, carotenoids, vitamins, and various mineral elements (Chudasama et al., 2022). Bioactive substances in seaweed mainly include polysaccharides, proteins, terpenoids, sterols, polyphenols, cyclic polysulfide compounds, macrolides, etc. Seaweeds mainly include red algae, green algae, brown algae, and microalgae. Red seaweed sulfated polysaccharides are mainly classified as agar and carrageenan.The polysaccharides of red algae are mainly composed of galactose with sulfate, and may contain small amounts of xylose and mannose. Ulvan is the main water-soluble polysaccharide in green algae, containing rhamnose, xylose, glucuronic acid and so on. There are three main types of fucoidan: fucoidan, fucoidan sulfate and fucoidan starch. Fucoidan in brown algae can be categorized into two main groups according to their sources: one group mainly comes from kelp, palm kelp, pine algae, branching tube algae, and cordyceps, etc.; the other group comes from the genera Vesiculoblastus and Murraya. Fucoidan has a complex structure, which not only has a variety of connection methods, but also contains monosaccharides such as fucose, a small amount of glucose, galactose, mannose, xylose and so on. Microalgae are a class of widely distributed, nutrient-rich, highly photosynthetically utilized autotrophic plants, and the products of cellular metabolism have good prospects for development in the fields of food, medicine, and genetic engineering(Barkia et al., 2019; Dolganyuk et al., 2020). Seaweed polysaccharides show superior bioactive diversity and good therapeutic potential, with antioxidant, antitumor, anti-inflammatory, antilipidemic, anticoagulant, antiviral, antibacterial, immunomodulatory, and other functional properties (Carson et al., 2018; Manlusoc et al., 2019; Cook et al., 2021). Current research has shown that the amount of specific secondary metabolites determines the potential for potent bioactivity of seaweeds also depend on the structural activity relationship of the compounds present in them, and that seaweed polysaccharides can reduce the risk of digestive disorders and chronic diseases, such as diabetes, cancer and cardiovascular disease, compared with other species of Seaweed, such as microSeaweed. Therefore, the incorporation of seaweed components into the production of novel natural medicines is one of the goals of marine medicine, a new discipline of pharmacology that has developed in recent decades. Some of these compounds have osteogenic potential, with such osteogenic compounds being dominated by the sulfated polysaccharides (SPs) of seaweed polysaccharides (Carson et al., 2018).
Regenerative medicine is an interdisciplinary discipline that has developed over the years and shown great promise in treating various diseases (Edgar et al., 2020). Studies have shown that seaweed polysaccharides can be effectively used in regenerative medicine (Chaves Filho et al., 2022), with bone tissue regeneration a popular research topic (Venkatesan et al., 2019). As research progresses, combining hydrogel, scaffold, and 3D printing technologies with seaweed polysaccharides becomes a new development trend. However, since few review articles cite the role of seaweed polysaccharides in bone tissue regeneration, this review focuses on their potential in bone regeneration. This review will introduce the main mechanisms of seaweed polysaccharides for bone regeneration and the novel preparations of seaweed polysaccharides, aiming to contribute to the regenerative medicine field.
2 Seaweed polysaccharides
The unique ecological environment of the ocean has led to the production of many biologically active substances with novel structures and diverse activities, the more notable being seaweed plant polysaccharides (Lomartire and Goncalves, 2022). Seaweed is one of the richest resources in the ocean, with high nutritional value, and has attracted significant attention (Chudasama et al., 2021). Polysaccharides are the main constituents of seaweeds, accounting for up to 76% of their dry weight (Xu et al., 2017). Polysaccharides are a structural component of the seaweed cell wall (de Jesus Raposo et al., 2015). Seaweed polysaccharides have good biological activity and have the advantages of being widely available, residue-free, and non-biotolerant (Echave et al., 2021). SP extracted from marine macroSeaweed can be used as a scaffolding system for the extracellular matrix of bone tissue and is usually closely associated with pharmacological activities such as anticoagulation, antioxidant, antitumor, and tissue regeneration (Saravana et al., 2018).
Seaweed SPs are a class of natural or chemically modified polysaccharides, a polysaccharide complex extracted from seaweed plants that can scavenge hydroxyl radicals and superoxide anion radicals and has the advantages of non-toxic side effects, inexpensive raw materials, easy to prepare, and easy to use (Beaumont et al., 2021). SPs have been shown to have specific biological activity in promoting bone tissue regeneration. These SPs are mainly divided into agarose and galactose, especially the 4-linked α-galactose residues of the l-series galactose called agarose and the d-series called galactose (Seedevi et al., 2017). In most seaweeds, the galactopyranosyl sulfate content is dominant, and galactopyranosyl sulfate has a linear backbone consisting of alternating 3-linked-D-galactopyranosyl units (A-unit) and 4-linked-galactopyranosyl units (B-unit) (Ciancia et al., 2020). SPs are classified as agarose and carrageenan according to their stereochemistry. Specifically, galactose with four attached α-galactose residues in the L series is called agarose, and galactose in the D series is called carrageenan. MacroSeaweed SPs have promising applications in building bone tissue repair and regeneration. For example, Kwack designed a fucoidan and polydopamine (PDA)-based composite membrane for use as a culture platform for periodontal ligament stem cells (PDLSCs) and explored the major molecular pathways induced during the osteogenic differentiation of PDLSCs by transcriptome profiling, showing that the fucoidan idan/PDA complex promotes the osteogenic potential of PDLSCs by activating key molecular pathways (Kwack et al., 2022) (Table 1).
Carrageenan is mainly derived from red seaweed, accounting for about 30%–75% of the dry weight of the seaweed cell wall (Qureshi et al., 2019). It is a natural polysaccharide carbohydrate polymer consisting of alternating d-galactose and 3,6-anhydrogalactose units (Ha et al., 2022) linked by α-1,3 and β-1,4-glycosidic bonds. More than 15 carrageenans have been reported to date (Thye et al., 2022). It is abundant in the extracellular matrix of red seaweeds (Usov, 2011). Carrageenan is an anionic sulfated polygalactose consisting of repeating disaccharide units with a sulfate content of 15%–40% and an average relative molecular mass >100,000 (Prajapati et al., 2014). Carrageenan has gained much attention in biomedical engineering, drug delivery, and biosensor applications in recent years due to its special properties, such as biocompatibility, biodegradability, and gel-forming ability (Li et al., 2014). Cao et al. Conducted a study in which they experimentally showed that κ-carrageenan promotes the adhesion and spreading, metabolic activity, proliferation, and osteogenic differentiation of MC3T3-E1 preosteoblasts, suggesting that κ-carrageenan is a potential osteogenic inducer for bone regeneration that could be used for clinical applications (Cao et al., 2021).
Fucoidan is an SP usually isolated from brown seaweed (Jayawardena et al., 2022). Kylin first isolated it in 1913 (Harada and Maeda, 1998). As a linear polysaccharide with different physiological and biochemical properties according to its structure and composition, fucoidan has great application value and potential in food, medicine, and cosmetics (Mabate et al., 2021). Fucoidan is a linear multimer consisting of β-D-mannuronate and α-L-guluronate units linked by 1→4 glycosidic bonds (Etman et al., 2020). Fucoidan can have different compositions and structures from different sources, such as a high fucoidan gulono-alkylate content (M/G residue ratio <1) in Laminaria hyperborea, low fucoidan gulono-alkylate content in marine macroSeaweed (Durvillaea potatorum), and acetylation in bacteria (Li et al., 2008; van Weelden et al., 2019). Fucoidan structure is also influenced by season, growth environment, age, and site in some brown Seaweed (Fitton et al., 2019; Tran et al., 2021). Small amounts of monosaccharides, such as glucose, xylose, mannose, and galactose, are also present in the molecular structure of fucoidan (Zayed et al., 2020).Fucoidan can induce bone formation in MG-63 cells and has also been shown to induce the osteogenic differentiation of stem cells for bone tissue regeneration (Venkatesan et al., 2015; Citkowska et al., 2019). Fucoidan has the molecular formula (C6H8O6)n and is a naturally biocompatible and biodegradable polymer widely used in various biomedical applications (Guo et al., 2020). Fucoidan is a natural linear polymeric. Alginate is present in the cell wall of brown Seaweed mainly as calcium alginate and partially as magnesium alginate, potassium alginate, and sodium alginate (SA) (Guo et al., 2020). Since fucoidan has good biocompatibility and antioxidation, gelation, and other properties, it is widely used in food, biomedicine, daily chemicals, textile printing and dyeing, and wastewater treatment (Dekamin et al., 2018). In bone tissue regeneration, fucoidan has osteogenic ability and promotes stem cell proliferation, resulting in sufficient osteoblast adhesion and thus accelerating the healing of bone tissue (Sikkema et al., 2021). In cartilage tissue engineering, it provides a microenvironment for chondrocyte growth, maintains the cell phenotype and its expression, and enables faster cartilage tissue healing (Huang et al., 2012).
Ulvan is the primary water-soluble polysaccharide found in green Seaweed. Like other polysaccharides found in seaweeds, its abundance in nature, wide range of sources, and high research value in medicine have made ulvan a hot research topic (Kikionis et al., 2022; Liu D. et al., 2022; Tanaka et al., 2022). Ulvan is a complex anionic SP of the genus Ulva, and studies of its biological activities have reported anticoagulant, antioxidant, antihyperlipidemic, antibacterial, antimicrobial, antiviral, tissue regenerative, and immunomodulatory properties. Several studies have found that ulvan can be used as a biomaterial polymer with excellent chemical properties, such as the easy hydrogel formation, scaffold formation, and electrospinning of nanofibers, which could potentially be used in biomedical applications (Ibrahim et al., 2022). It has unique functional properties compared to other seaweed polysaccharides, such as carboxyl, hydroxyl, and sulfate groups (Lakshmi et al., 2020). The main component of ulvan’s chemical structure is sulfated rhamnose, which is linked to uronic acid (glucuronide and/or iduronic acid) by a 1,4-glycosidic bond (Sulastri et al., 2021). Ulvan’s biosynthesis and, therefore, its chemical structure vary by seaweed species, harvesting areas, and growing conditions (Ahmad et al., 2021). These large differences in chemical structure lead to differences in physiological functions, although the exact mechanisms of action remain unclear.
The unique ecological environment of the ocean has led to the production of many biologically active substances with novel structures and diverse activities, the more notable of which are seaweed polysaccharides. Their diverse and heterogeneous structure makes studying their structure challenging and may hinder their development as therapeutic agents. Due to their chemical and biological properties, seaweed SPs have great potential for biomedical applications, especially in bone tissue regeneration. Seaweed polysulfates have promising applications in promoting bone tissue repair and regeneration.
3 Application of seaweed polysaccharides in bone regeneration
Bone tissue regeneration undergoes three ongoing and overlapping phases: inflammation, regeneration, and remodeling. Initial inflammation induces immune cells such as T cells or monocytes. These cells produce cytokines such as tumor necrosis factor (TNF) and interleukin (IL)-6. However, infection may lead to prolonged inflammation, and systemic circulation of the remaining pro-inflammatory cytokines, such as IL-6, TNF, and IL-1, can adversely affect bone regeneration (Newman et al., 2021). Seaweed polysaccharides can induce osteogenic differentiation of stem/progenitor cells (Chaves Filho et al., 2022). It can also exert its anti-inflammatory effects by modulating a series of inflammation-related signaling pathways, including NF-κB, MAPK, JAK/STAT, PI3K/AKT, and Nrf2/HO-1, thereby regulating the production of various inflammation-related factors. Macrophages are an important target for bone repair in bone tissue engineering (Sadowska and Ginebra, 2020). Building a locally appropriate bone immune microenvironment is a novel therapeutic strategy (Schlundt et al., 2021; Healy et al., 2023). Macrophages secrete cells that regulate inflammation and control the function of osteoblasts. M1 macrophages secrete inflammatory cytokines, including TNF, IL-1β, IL-6, IL-12, and IL-23. M2 macrophages release anti-inflammatory cytokines, including IL-10, transforming growth factor (TGF)-β, and IL-1RA. One study on IL-10-deficient mice showed that IL-10 enhances osteoblast differentiation. TGF-β is an osteogenic factor upstream of the bone morphogenetic protein (BMP) signaling pathway that ultimately induces osteoblast differentiation (Lee et al., 2019). Factors such as TGF-β and IL-4 induce osteoblast migration, proliferation, or bone extracellular matrix secretion in the early stages of differentiation (Apostolova et al., 2020) (Figure 1).
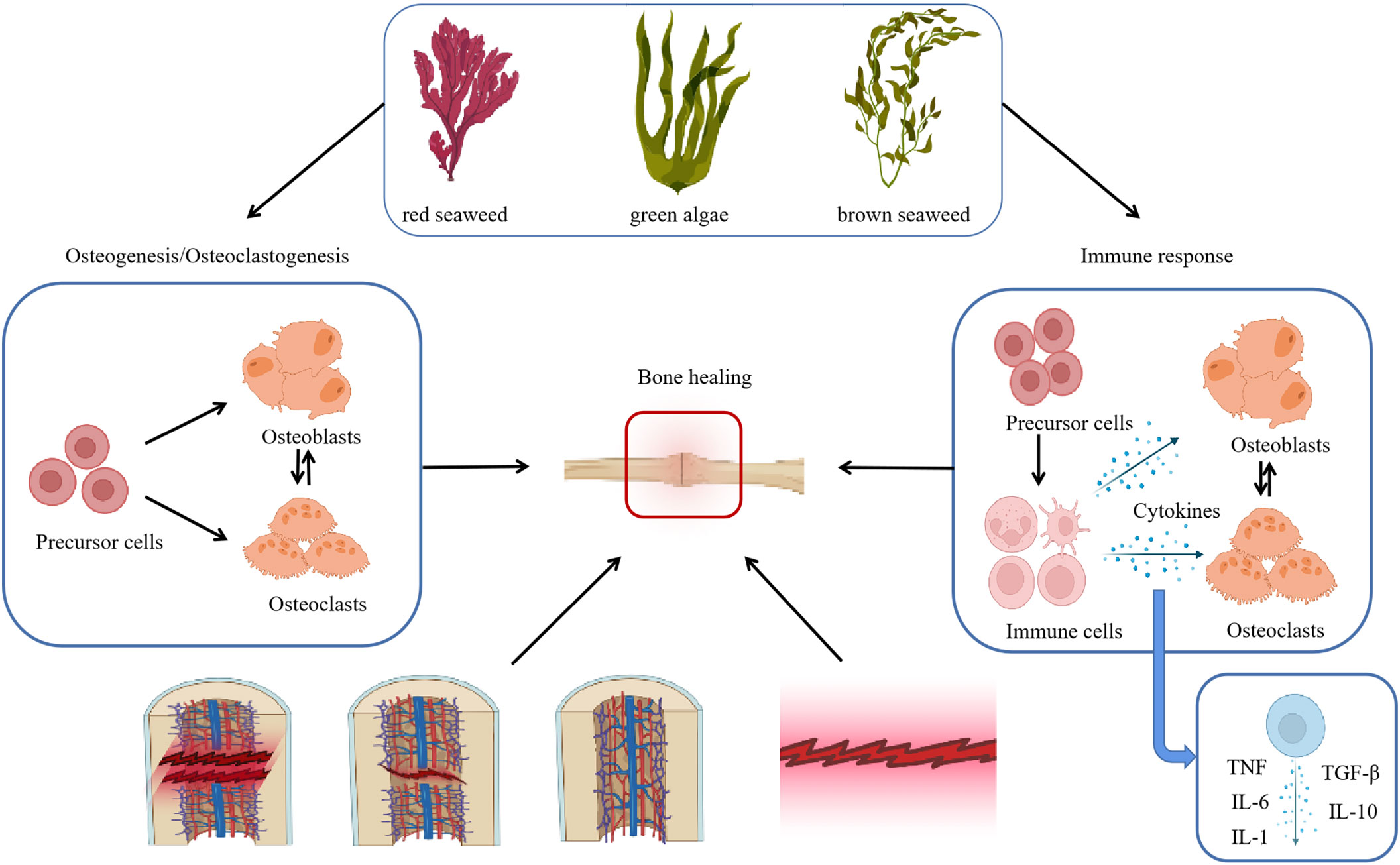
Figure 1 Some cells in seaweed polysaccharide act on bone healing. Osteoblasts and osteoclasts in different species of seaweed can act in the process of bone healing. Osteoblasts and osteoclasts promote bone healing by acting as an immune response to broken bone tissue through cytokines secreted by immune cells. TNF(Tumor necrosis factor) TGF-β(Transforming growth factor-β) IL-6(interleukin 6) IL-1(interleukin 1 ) IL-10(interleukin 10).
Alginate significantly affects the phenotype and function of macrophages (Sadowska and Ginebra, 2020; Li et al., 2023c). Alginate is the most commonly used polysaccharide and stimulates cartilage regeneration (Popa et al., 2015). Alginate is an anionic biopolymer derived from brown Seaweed with a structure similar to glycosaminoglycans in the extracellular matrix. Simulation of gelatin and alginate as collagen and glycosaminoglycan in the extracellular matrix, respectively (Valcarcel et al., 2017) creates hydrogels with excellent mechanical properties, swelling stability, biodegradability, and biocompatibility, meeting the requirements of tissue regeneration (Taghipour et al., 2020; Ahmad Raus et al., 2021). Hydrogel compounded with osteopontin significantly promoted bone regeneration when used in a critical-size bone defect rat model (Wu et al., 2023). A SA/G/MXene composite membrane was prepared by combining the sol-gel and freeze-drying methods. Adding SA/G/MXene improved the SA/G membranes’ mechanical and hydrophilic properties and cell proliferation and osteogenic differentiation (Qin et al., 2023).
Alginate can be introduced into the body through minimally invasive techniques. Because alginate gels lack the proper mechanical strength to withstand the initial stages of regeneration without fixation, studies have demonstrated that they can deliver growth factors and thus effectively promote bone regeneration (Kothale et al., 2020). The controlled release of vascular endothelial growth factor (VEGF) molecules encapsulated in alginate particles in a collagen-hydroxyapatite (HAP) scaffold promoted post-implantation scaffold angiogenesis (Quinlan et al., 2017). The researchers tested bone healing with ethyl triacetate and tributyl citrate plasticized alginate. In vivo studies have shown alginate and triacetate-modified scaffolds to have a growth-promoting effect on osteoblasts (Arslan et al., 2023). Alginate shows promise in bone tissue engineering and regenerative medicine, and it can also inform drug/growth factor delivery therapeutic strategies for diseases requiring specific drug/growth factor duration of action (Zhao et al., 2022).
Seaweed SPs have a role in promoting or enhancing osteogenesis, which is closely related to osteoblast differentiation. SP extracted from marine Seaweed can be used to construct extracellular bone tissue matrix scaffold systems. In addition, SPs have been shown to have osteoinductive properties, which are important for developing artificial bone tissue. There will also be differences in the form of polysulfate scaffold systems, such as hydrogels, composites, blends, nanoparticles, electrostatically spun fibers, and 3D printed materials (Venkatesan et al., 2019; Hakimi et al., 2023). SPs significantly affected recombinant human BMP-2-induced bone regeneration, with enhanced bone regeneration-promoting ability in both in vivo and in vitro experiments.
Fucoidan is a naturally occurring active polysaccharide rich in sulfate groups produced by brown Seaweed (Chollet et al., 2016; Li et al., 2023a). Its slow and sustained release positively affects osteogenic differentiation (Carson and Clarke, 2018; Yadav and Song, 2022). Researchers have developed an alginate-nano HAP-nano graphene oxide carrier of rockweed polysaccharide as an osteoinductive scaffold to repair and regenerate bone tissue (Devi et al., 2022; Ohmes et al., 2022). The natural rockweed polysaccharide (Fuc) extracted from brown Seaweed was coupled with gelatin (gel) to form a template to prepare a bionic scaffold. The Fuc-Gel-MTN peptide (MTNYDEAAMAIASLN)-β-tricalcium phosphate/HAP scaffold could promote bone formation and mineralization, and studies have shown that it has a very high potential for bone tissue regeneration (Pajovich and Banerjee, 2017; Nunes and Coimbra, 2019). Fucoidan and PDA were used as carriers to construct an in vitro culture system for PDLSCs. The main molecular pathways induced during PDLSC osteogenic differentiation were explored by transcriptomic profiling, and the key signaling pathways that play a critical role in PDLSC osteogenic differentiation were screened. Their complexes promote the osteogenic potential of PDLSCs by activating key molecular pathways (Kwack et al., 2022; Nielsen et al., 2022).
Bone is highly vascularized. Growth factors such as VEGF and BMP-2 are involved in angiogenesis (Liu et al., 2015). These angiogenic factors can bind directly or indirectly to polymers through covalent interactions or ligand-based adsorption, respectively. Studies have reported that VEGF gene expression and protein secretion were significantly increased with the promotion of osteogenic differentiation by rockweed polysaccharides and that VEGF/VEGF receptor inhibitors significantly inhibited VEGF’s vasculogenic effect. One study found that the medium of mesenchymal stem cell (MSC) conditioned with rockweed polysaccharide upregulated the phosphorylation of phosphoinositide 3-kinase (PI3K), protein kinase B (AKT), endothelial nitric oxide synthase (eNOS) to inhibit its pro-angiogenic effect via the AKT/eNOS pathway. Animal experiments showed that rockweed polysaccharide has significant promotive effects on cranial defects and neovascularization in rabbits (Kim et al., 2018).
Kappa carrageenan (κ-CG) and ulvan have only recently been featured in some reports. Carrageenan is an SP extracted from red seaweed (Prajapati et al., 2014). It is highly hydrophilic and biocompatible and enhances the activity of the pre-osteoblastic cells required for bone regeneration (Yegappan et al., 2018; Cao et al., 2021). Researchers found that κ-CG influenced the morphology and microstructure of the octacalcium phosphate coating on titanium discs, resulting in increased preosteoblast metabolic activity, proliferation, and osteogenic differentiation (Cao et al., 2022). A series of electrospun silk fiber (SF)/κ-CG nanofiber membranes were used to mimic the structure and composition of the bone extracellular matrix to improve the biological properties of SF-based nanofibers. The results show that combining κ-CG and SF could effectively improve the biological properties of nanostructured scaffolds. κ-CG also enhanced the SF nanofibers’ osteogenic potential and bioactivity (Roshanfar et al., 2022). A potassium chloride cross-linked 3D scaffold composed of κ-CG, chitosan, and gelatin was evaluated for its mechanical and biological properties in bone tissue engineering. All materials showed good biocompatibility and a high differentiation potential with MC3T3-E1 preosteoblasts (Loukelis et al., 2022).
Ulvan, a biologically active marine SP, is of interest for its good osteoinductive properties (Tziveleka et al., 2019). Because of the lack of systematic studies on the relationship between the conformation and properties of ulvan-like compounds, their specific relevance is unclear (Tziveleka et al., 2019). Ulvan’s osteoinductive ability meant that when it was incorporated into a polycaprolactone matrix, it effectively improved cell attachment and viability, demonstrating its potential for bone tissue regeneration applications in biomedical scaffolds (Kikionis et al., 2021). The hybrid sponge-like scaffold prepared by inoculating human adipose-derived MSCs into selected ulvan/gelatin hybrid scaffolds could effectively support MSC adhesion and proliferation, making it a promising new bone tissue engineering material (Tziveleka et al., 2020). Ulvan’s important bioactivity and tunable physicochemical and rheological properties have made it of interest as a novel composite biomedical material (Table 2).
Researchers have shown that the seaweed component SP has favorable osteogenic activity. In fact, most of the in vitro experiments have shown that SP has osteogenesis-promoting effects, but only two experiments have investigated the function of SP in vivo, so further experiments are needed to verify this. In addition, since the in vitro environment does not fully mimic the situation in living organisms, many cell types and systems are osteogenic-promoting. Therefore, the study of osteogenesis by in vivo experiments or using more complex animal models is an important part of the process.
4 New dosage forms loaded with seaweed polysaccharides
Seaweeds are a large group of low-level photosynthetic autotrophs distributed in various seas and coastal zones worldwide. They have become a hot spot for research globally because they contain various bioactive components. Preparing new polysaccharide dosage forms with suitable carriers will help reduce adverse drug reactions, improve the therapeutic index, and enhance targeting, making exploring seaweed polysaccharide dosage forms important.
4.1 Hydrogel loaded with seaweed polysaccharides
Natural polymer hydrogels have the advantages of good biocompatibility, regeneration, and low immune rejection (Huang et al., 2017). Alginate hydrogel is a special type of hydrogel extracted and transformed from seaweed and is an attractive material for cellular microencapsulation (Lertwimol et al., 2022; Wang et al., 2022). SA has the characteristics of other easy-to-gel hydrogels and the advantages of good biocompatibility, degradability, and non-toxicity. Numerous studies have shown that ionic cross-linking with divalent cations (e.g., Ca2+) to prepare hydrated gels is a new biomaterial with very promising applications (Labowska et al., 2023). SA/hydroxyethylcellulose/HAP porous scaffolds with pore sizes larger than 89.5 ± 4.58 μm were prepared. The results showed that the compounding of hyaluronic acid (HA) enhanced the mechanical properties of the composite scaffold material and improved its biocompatibility and bioactivity (Tohamy et al., 2018). The researchers developed biocompatible nanogel/hydrogel nanocomposites and introduced pH, thermal, and magnetic response properties into them as a sustained release drug carrier for levodopa, developing a biocompatible hydrogel/nanocomposite system for long-lasting delivery of biologically active substances (Bardajee et al., 2020; Gan et al., 2023).
Carrageenan/chitosan hydrogels with physical properties are constructed by electrostatically combining carrageenan with chitosan, which have different charging properties. The composite hydrogel maintains its mechanical properties in the physiological pH range, showing the potential of the prepared hydrogel as a multifunctional biomaterial for drug delivery, tissue engineering, and bone repair (Papagiannopoulos et al., 2023). Researchers prepared injectable sprayable hydrogels based on visible light cross-linked methacrylic acid κ-CG with high gel strength, low weight loss, and high water retention capacity to maintain cell apposition and proliferation in vitro (Tavakoli et al., 2019; Moncada et al., 2023). HA is widely used in tissue engineering because of its good biocompatibility and degradability. HA and rockweed polysaccharide form a composite hydrogel with a flexible structure and good ecological position for inducing osteogenic differentiation, making it a new bone repair material with very promising applications (Amin et al., 2021; Hsu et al., 2021; Abouzeid et al., 2022). Trimethoprim-loaded chitosan/fucoidan composite hydrogels were prepared by chemical cross-linking method (Amiri et al., 2022; Carvalho et al., 2022). Adding fucoidan to the composite hydrogel significantly improved its swelling properties, mechanical strength, and adhesion properties (Wang et al., 2021; Zheng et al., 2021).
Despite the promising combination of seaweed polysaccharides and hydrogels, some challenges remain in their clinical application. Currently, most systems are studied in vitro, and their in vivo mechanisms of action remain unclear. Therefore, future studies should gradually shift to in vivo experiments.
4.2 Seaweed polysaccharide scaffolds
Because of their excellent biocompatibility, calcium alginate hydrogels are widely used in the study of tissue engineering scaffold materials (Chaudhari et al., 2016). The scaffold materials modulate cell adhesion using arginine-glycine-aspartic acid (RGD) peptides grafted onto alginate. In vitro cellular experiments have shown that RGD grafting can positively affect osteogenic differentiation and bone tissue repair (Ingavle et al., 2019). The gel material has good surface adhesion and facilitates cell adhesion, proliferation, and differentiation. It is also a highly porous structure that can provide cell adhesion and stimulate new tissue growth. Using oxidized SA complexed with borax and then reacted with Schiff bases and self-crosslinked with gelatin resulted in an injectable hydrogel to repair meniscal tissue. Chondrocytes can adhere and proliferate on the surface of this composite hydrogel, and there is good integration between the scaffold material and meniscal tissue (Resmi et al., 2020).
Carrageenan is widely used as an injectable scaffold material due to its superior properties (Sairaman et al., 2022). Different κ-CG concentrations were first mixed with filaggrin (FLG) to make a composite gel, and then the scaffold was prepared by freeze-drying to mimic the extracellular matrix component of bone, which has an interconnected and highly porous structure. The scaffold could promote human osteogenic sarcoma cell proliferation and increase their alkaline phosphatase (ALP) activity, which can potentially mimic the bone’s extracellular matrix component (Nourmohammadi et al., 2017). κ-CG sulfate was added to scaffolds to affect bone matrix mineralization and cell adhesion (Muscolino et al., 2022a), demonstrating the prospect of collagen/κ-CG scaffolds as biomaterials. Different ratios of polyvinyl alcohol and κ-CG were mixed using a cryogel technology to obtain 3D, interconnected, highly porous, biodegradable scaffolds. The gel scaffold had high compatibility with hemoglobin, and its scaffold material also had excellent characteristics for tissue engineering applications and long-term cell cryopreservation (Chopra et al., 2016; Aslam Khan et al., 2021).
Rockweed polysaccharides can form stable 3D structures with other polymers and, therefore, are used to develop delivery systems or tissue regeneration scaffolds (Nunes and Coimbra, 2019). An osteoinductive scaffold derived from diatom-derived alglucosidase compounded with SA-nanoapatite-nano-graphene oxide could be used as a bone graft substitute (Lu et al., 2019; Bharadwaz and Jayasuriya, 2020; Devi et al., 2022). Polyether carbonate urea nanofiber scaffolds loaded with the natural marine bioactive rock Seaweed polysaccharide have been studied and found to have potential applications (Carvalho et al., 2022; Yu et al., 2022).
4.3 3D printing with seaweed polysaccharides
Seaweed can produce a wide range of polymers with properties meeting the requirements of 3D printing and unique prospects for use as biomaterials (Mandal et al., 2023). 3D bioprinting can mimic the physical, chemical, and biological laws of nature to provide a bionic structural environment for tissue generation and host integration, enabling the use of living cells to build complex tissue structures (Mahendiran et al., 2021; Fatimi, 2022). Its principle is simply to add a 3D plane to the ordinary 2D plane. Like ordinary printers, 3D printers use plastic, metal, or other powdered materials to print out a single layer. Then, additional layers are progressively added to form a 3D object (Li et al., 2023b). 3D bioprinting technology enables rapid fabrication of complex 3D cell-loaded scaffolds for tissue engineering applications (Kumari et al., 2022). Alginate/G/cellulose nanocrystal composite hydrogels were prepared using 3D printing technology, showing higher mechanical strength than single-component polymer scaffolds. In animal studies, this hydrogel helped to improve bone regeneration potential (Dutta et al., 2021; Zineh et al., 2022). Bacterial cellulose was incorporated into an alginate/bacterial cellulose nanocrystals-chitosan- gelatin composite gelatin scaffold, which was shown to have a good 3D structure (Abbasi-Ravasjani et al., 2022). In vitro experiments showed that human osteoma and mouse cells exhibited good pore structure on a composite gel scaffold, among other advantages (Li et al., 2021).
Carrageenan is very promising as a 3D printing bio-ink material in tissue engineering and regenerative medicine (Liu et al., 2023). Hydrogels were prepared from κ-CG methacrylate, in which mouse embryonic cells were encapsulated. The cell-loaded hydrogels showed cytocompatibility and retained cell morphology (Lim et al., 2020). 3D printing hydrogels using hybrid hydrogel inks (polyvinyl alcohol and k-angle gum) with excellent rheological properties and a strong affinity for cells allowed cells to bind tightly to the hydrogel surface (Jiang et al., 2019; Muscolino et al., 2022b). 3D porous scaffolds have been modified with a biosynthetic alginate-sulfate base to slowly release the VEGF for its pro-angiogenic activity (Liu Y. et al., 2022).
In an in vitro assay, rockweed polysaccharide significantly promoted endothelial progenitor cells in microporous and macroporous scaffolds (Purnama et al., 2015). Scaffolds created from ulvan methacrylate and methacryloyl Gel had mechanics, structures, and bioactivities that could reach a similar level to human skin and had better cytocompatibility (Chen et al., 2021). Therefore, a new bionic ink with both shear thinning, yield stress, and tunable mechanical properties was prepared to realize the bionic printing of 3D porous structures on the surface of bionic materials. Ulvan, a biomaterial for 3D printing, has poor rheological properties and has difficulty solubilizing in various solution systems (Mahendiran et al., 2021).
However, many technical difficulties remain to be overcome to realize the clinical application of this technology and to provide technical assistance for future multidisciplinary printing. For example, the geometries and microstructures of scaffolds made with currently available materials do not yet meet the requirements for clinical applications. Zhang et al. believe that integrating biochemical molecules directly into 3D-printed scaffolds may enhance their microstructure and ultimately accelerate bone regeneration at the defect site (Zhang et al., 2019) (Table 3).
5 Conclusions and outlook
The ocean contains many compounds with a wide range of applications in biomedicine and biotechnology. It is a major renewable source of natural substances, further driving the development of new medical systems and devices (Sharma et al., 2023). In marine systems, macroSeaweed are an important source of primary and secondary metabolites of biological importance (Ren et al., 2022). Brown Seaweed contain the most compounds, followed by green and red Seaweed (Alghazeer et al., 2022). In recent years, the species and structures of seaweeds and seaweed metabolites have been intensively studied in the hope of discovering new bioactive compounds. Seaweed SPs have good potential for exploitation as bioactive substances (Ngo and Kim, 2013; Arokiarajan et al., 2022). Rockweed polysaccharides and carrageenan are two important types of seaweed SPs with a wide range of biological properties (Oliyaei et al., 2022).
Seaweed polysaccharides are considered a potential biomedical material due to their unique chemical and biological properties (Walsh et al., 2019). Carrageenan from red Seaweed, rockweed polysaccharide from brown Seaweed, and ulvan from green Seaweed show good biocompatibility. They could be used as scaffolds, nanofibers, hydrogels, or other biocompatible materials, which are expected to become a new generation of bone repair materials (Pajovich and Banerjee, 2017; Venkatesan et al., 2019). With advancements in orthopedic technology and improvements in biomaterial properties, bone tissue regeneration has been realized (Lopes et al., 2018; Ansari, 2019). Seaweed polysaccharides have promising applications in treating or constructing bone tissue repair and regeneration (Chaves Filho et al., 2018). However, few studies have explored seaweed polysaccharides such as SP in the bone tissue engineering field. In response to this situation, they should be systematically studied in the future using animal research methods with large samples (Veronesi et al., 2020). Stem cell therapy for osteoporosis is currently a hot topic, but its mechanism of action remains unclear. Combining seaweed polysaccharides with stem cells is expected to be a new biomaterial with promising applications. 3D tissue or cell cultures based on seaweed polysaccharides are also being explored, and these materials could be applied in areas such as drug evaluation, complex cell physiology, and tissue engineering (Jain et al., 2018).
Author contributions
Conceptualization: YJ and SL. Methodology: QY. Software: TC. Validation: YJ, DL and TC. Formal analysis: QY. Investigation: SL. Resources: YJ. Data curation: DL. Writing—original draft preparation: YJ. Writing—review and editing: TC. Visualization: QY. Supervision: SL. Project administration: YJ. Funding acquisition: DL. All authors have read and agreed to the published version of the manuscript.
Funding
This research was funded by the National Natural Science Foundation of China (Grant Nos 82003985 and 81973712), China Postdoctoral Science Foundation (Grant Nos 2020M670825 and 2020T130568), Jilin Province Science and Technology Development Project in China (Grant Nos 20210101192JC, 20210204013YY, and 20200504005YY), National College Students’ innovation and entrepreneurship training program (Grant Nos 202210199004 and 202210199011X).
Conflict of interest
The authors declare that the research was conducted in the absence of any commercial or financial relationships that could be construed as a potential conflict of interest.
Publisher’s note
All claims expressed in this article are solely those of the authors and do not necessarily represent those of their affiliated organizations, or those of the publisher, the editors and the reviewers. Any product that may be evaluated in this article, or claim that may be made by its manufacturer, is not guaranteed or endorsed by the publisher.
References
Abbasi-Ravasjani S., Seddiqi H., Moghaddaszadeh A., Ghiasvand M. E., Jin J., Oliaei E., et al. (2022). Sulfated carboxymethyl cellulose and carboxymethyl kappa-carrageenan immobilization on 3D-printed poly-epsilon-caprolactone scaffolds differentially promote pre-osteoblast proliferation and osteogenic activity. Front. Bioeng Biotechnol. 10. doi: 10.3389/fbioe.2022.957263
Abouzeid R. E., Salama A., El-Fakharany E. M., Guarino V. (2022). Mineralized polyvinyl alcohol/sodium alginate hydrogels incorporating cellulose nanofibrils for bone and wound healing. Molecules 27 (3), 697. doi: 10.3390/molecules27030697
Ahmad T., Eapen M. S., Ishaq M., Park A. Y., Karpiniec S. S., Stringer D. N., et al. (2021). Anti-inflammatory activity of fucoidan extracts in vitro. Mar. Drugs 19 (12), 702. doi: 10.3390/md19120702
Ahmad Raus R., Wan Nawawi W. M. F., Nasaruddin R. R. (2021). Alginate and alginate composites for biomedical applications. Asian J. Pharm. Sci. 16 (3), 280–306. doi: 10.1016/j.ajps.2020.10.001
Alazzam A. M., Goldsmith J. A., Khalil R. E., Khan M. R., Gorgey A. S. (2023). Denervation impacts muscle quality and knee bone mineral density after spinal cord injury. Spinal Cord 61 (4), 276–284. doi: 10.1038/s41393-023-00885-3
Alghazeer R., El Fatah H., Azwai S., Elghmasi S., Sidati M., El Fituri A., et al. (2022). Nutritional and nonnutritional content of underexploited edible seaweeds. Aquac Nutr. 2022, 8422414. doi: 10.1155/2022/8422414
Amin M. L., Mawad D., Dokos S., Koshy P., Martens P. J., Sorrell C. C. (2021). Fucoidan- and carrageenan-based biosynthetic poly(vinyl alcohol) hydrogels for controlled permeation. Mater Sci. Eng. C Mater Biol. Appl. 121, 111821. doi: 10.1016/j.msec.2020.111821
Amiri S., Asghari A., Harifi-Mood A. R., Rajabi M., He T., Vatanpour V. (2022). Polyvinyl alcohol and sodium alginate hydrogel coating with different crosslinking procedures on a PSf support for fabricating high-flux NF membranes. Chemosphere 308 (Pt 2), 136323. doi: 10.1016/j.chemosphere.2022.136323
Ansari M. (2019). Bone tissue regeneration: biology, strategies and interface studies. Prog. Biomater 8 (4), 223–237. doi: 10.1007/s40204-019-00125-z
Apostolova E., Lukova P., Baldzhieva A., Katsarov P., Nikolova M., Iliev I., et al. (2020). Immunomodulatory and anti-inflammatory effects of fucoidan: A review. Polymers (Basel) 12 (10), 2338. doi: 10.3390/polym12102338
Arokiarajan M. S., Thirunavukkarasu R., Joseph J., Ekaterina O., Aruni W. (2022). Advance research in biomedical applications on marine sulfated polysaccharide. Int. J. Biol. Macromol 194, 870–881. doi: 10.1016/j.ijbiomac.2021.11.142
Arslan A. K., Aydogdu A., Tolunay T., Basat C., Bircan R., Demirbilek M. (2023). The effect of alginate scaffolds on bone healing in defects formed with drilling model in rat femur diaphysis. J. BioMed. Mater Res. B Appl. Biomater. 111 (6), 1299–1308. doi: 10.1002/jbm.b.35233
Aslam Khan M. U., Raza M. A., Mehboob H., Abdul Kadir M. R., Abd Razak S. I., Shah S. A., et al. (2021). Correction: Development and in vitro evaluation of kappa-carrageenan based polymeric hybrid nanocomposite scaffolds for bone tissue engineering. RSC Adv. 11 (30), 18615–18616. doi: 10.1039/d1ra90119b
Bardajee G. R., Khamooshi N., Nasri S., Vancaeyzeele C. (2020). Multi-stimuli responsive nanogel/hydrogel nanocomposites based on kappa-carrageenan for prolonged release of levodopa as model drug. Int. J. Biol. Macromol 153, 180–189. doi: 10.1016/j.ijbiomac.2020.02.329
Barkia I., Saari N., Manning S. R. (2019). Microalgae for high-value products towards human health and nutrition. Mar. Drugs 17 (5), 304. doi: 10.3390/md17050304
Beaumont M., Tran R., Vera G., Niedrist D., Rousset A., Pierre R., et al. (2021). Hydrogel-forming algae polysaccharides: from seaweed to biomedical applications. Biomacromolecules 22 (3), 1027–1052. doi: 10.1021/acs.biomac.0c01406
Bharadwaz A., Jayasuriya A. C. (2020). Recent trends in the application of widely used natural and synthetic polymer nanocomposites in bone tissue regeneration. Mater Sci. Eng. C Mater Biol. Appl. 110, 110698. doi: 10.1016/j.msec.2020.110698
Cao W., Jin J., Wu G., Bravenboer N., Helder M. N., Pathak J. L., et al. (2021). K-carrageenan stimulates pre-osteoblast proliferation and osteogenic differentiation: A potential factor for the promotion of bone regeneration? Molecules 26 (20), 6131. doi: 10.3390/molecules26206131
Cao W., Jin J., Wu G., Bravenboer N., Helder M. N., Schulten E., et al. (2022). Kappa-carrageenan-Functionalization of octacalcium phosphate-coated titanium Discs enhances pre-osteoblast behavior and osteogenic differentiation. Front. Bioeng Biotechnol. 10. doi: 10.3389/fbioe.2022.1011853
Carson M. A., Clarke S. A. (2018). Bioactive compounds from marine organisms: potential for bone growth and healing. Mar. Drugs 16 (9), 340. doi: 10.3390/md16090340
Carson M. A., Nelson J., Cancela M. L., Laize V., Gavaia P. J., Rae M., et al. (2018). Screening for osteogenic activity in extracts from Irish marine organisms: The potential of Ceramium pallidum. PloS One 13 (11), e0207303. doi: 10.1371/journal.pone.0207303
Carvalho D. N., Williams D. S., Sotelo C. G., Perez-Martin R. I., Mearns-Spragg A., Reis R. L., et al. (2022). Marine origin biomaterials using a compressive and absorption methodology as cell-laden hydrogel envisaging cartilage tissue engineering. Biomater Adv. 137, 212843. doi: 10.1016/j.bioadv.2022.212843
Chaudhari A. A., Vig K., Baganizi D. R., Sahu R., Dixit S., Dennis V., et al. (2016). Future prospects for scaffolding methods and biomaterials in skin tissue engineering: A review. Int. J. Mol. Sci. 17 (12), 1974. doi: 10.3390/ijms17121974
Chaves Filho G. P., de Sousa A. F. G., Camara R. B. G., Rocha H. A. O., de Medeiros S. R. B., Moreira S. M. G. (2018). Genotoxicity and osteogenic potential of sulfated polysaccharides from Caulerpa prolifera seaweed. Int. J. Biol. Macromol 114, 565–571. doi: 10.1016/j.ijbiomac.2018.03.132
Chaves Filho G. P., Lima M., Rocha H. A. O., Moreira S. M. G. (2022). Role of sulfated polysaccharides from seaweeds in bone regeneration: A systematic review. Carbohydr Polym 284, 119204. doi: 10.1016/j.carbpol.2022.119204
Chen X., Yue Z., Winberg P. C., Lou Y. R., Beirne S., Wallace G. G. (2021). 3D bioprinting dermal-like structures using species-specific ulvan. Biomater Sci. 9 (7), 2424–2438. doi: 10.1039/d0bm01784a
Chollet L., Saboural P., Chauvierre C., Villemin J. N., Letourneur D., Chaubet F. (2016). Fucoidans in nanomedicine. Mar. Drugs 14 (8), 145. doi: 10.3390/md14080145
Chopra P., Nayak D., Nanda A., Ashe S., Rauta P. R., Nayak B. (2016). Fabrication of poly(vinyl alcohol)-Carrageenan scaffolds for cryopreservation: Effect of composition on cell viability. Carbohydr Polym 147, 509–516. doi: 10.1016/j.carbpol.2016.04.027
Chudasama N. A., Polisetti V., Maity T. K., Reddy A. V. R., Prasad K. (2022). Preparation of seaweed polysaccharide based hydrophobic composite membranes for the separation of oil/water emulsion and protein. Int. J. Biol. Macromol 199, 36–41. doi: 10.1016/j.ijbiomac.2021.12.087
Chudasama N. A., Sequeira R. A., Moradiya K., Prasad K. (2021). Seaweed polysaccharide based products and materials: an assessment on their production from a sustainability point of view. Molecules 26 (9), 2608. doi: 10.3390/molecules26092608
Ciancia M., Matulewicz M. C., Tuvikene R. (2020). Structural diversity in galactans from red seaweeds and its influence on rheological properties. Front. Plant Sci. 11. doi: 10.3389/fpls.2020.559986
Citkowska A., Szekalska M., Winnicka K. (2019). Possibilities of fucoidan utilization in the development of pharmaceutical dosage forms. Mar. Drugs 17 (8), 458. doi: 10.3390/md17080458
Cook B., Walker N., Zhang Q., Chen S., Evans T. (2021). The small molecule DIPQUO promotes osteogenic differentiation via inhibition of glycogen synthase kinase 3-beta signaling. J. Biol. Chem. 296, 100696. doi: 10.1016/j.jbc.2021.100696
de Jesus Raposo M. F., de Morais A. M., de Morais R. M. (2015). Marine polysaccharides from algae with potential biomedical applications. Mar. Drugs 13 (5), 2967–3028. doi: 10.3390/md13052967
Dekamin M. G., Karimi Z., Latifidoost Z., Ilkhanizadeh S., Daemi H., Naimi-Jamal M. R., et al. (2018). Alginic acid: A mild and renewable bifunctional heterogeneous biopolymeric organocatalyst for efficient and facile synthesis of polyhydroquinolines. Int. J. Biol. Macromol 108, 1273–1280. doi: 10.1016/j.ijbiomac.2017.11.050
Devi G. V. Y., Nagendra A. H., Shenoy P. S., Chatterjee K., Venkatesan J. (2022). Fucoidan-incorporated composite scaffold stimulates osteogenic differentiation of mesenchymal stem cells for bone tissue engineering. Mar. Drugs 20 (10), 589. doi: 10.3390/md20100589
Dolganyuk V., Belova D., Babich O., Prosekov A., Ivanova S., Katserov D., et al. (2020). Microalgae: A promising source of valuable bioproducts. Biomolecules 10 (8), 1153. doi: 10.3390/biom10081153
Dutta S. D., Hexiu J., Patel D. K., Ganguly K., Lim K. T. (2021). 3D-printed bioactive and biodegradable hydrogel scaffolds of alginate/gelatin/cellulose nanocrystals for tissue engineering. Int. J. Biol. Macromol 167, 644–658. doi: 10.1016/j.ijbiomac.2020.12.011
Echave J., Fraga-Corral M., Garcia-Perez P., Popovic-Djordjevic J., Radulovic M., Xiao J., et al. (2021). Seaweed protein hydrolysates and bioactive peptides: extraction, purification, and applications. Mar. Drugs 19 (9), 500. doi: 10.3390/md19090500
Edgar L., Pu T., Porter B., Aziz J. M., La Pointe C., Asthana A., et al. (2020). Regenerative medicine, organ bioengineering and transplantation. Br. J. Surg. 107 (7), 793–800. doi: 10.1002/bjs.11686
Etman S. M., Elnaggar Y. S. R., Abdallah O. Y. (2020). Fucoidan, a natural biopolymer in cancer combating: From edible algae to nanocarrier tailoring". Int. J. Biol. Macromol 147, 799–808. doi: 10.1016/j.ijbiomac.2019.11.191
Fatimi A. (2022). Exploring the patent landscape and innovation of hydrogel-based bioinks used for 3D bioprinting. Recent Adv. Drug Delivery Formul 16 (2), 145–163. doi: 10.2174/2667387816666220429095834
Fitton H. J., Stringer D. S., Park A. Y., Karpiniec S. N. (2019). Therapies from fucoidan: new developments. Mar. Drugs 17 (10), 571. doi: 10.3390/md17100571
Fu R., Bertrand D., Wang J., Kavaseri K., Feng Y., Du T., et al. (2022). In vivo and in silico monitoring bone regeneration during distraction osteogenesis of the mouse femur. Comput. Methods Programs BioMed. 216, 106679. doi: 10.1016/j.cmpb.2022.106679
Gan X., Li C., Sun J., Zhang X., Zhou M., Deng Y., et al. (2023). GelMA/kappa-carrageenan double-network hydrogels with superior mechanics and biocompatibility. RSC Adv. 13 (3), 1558–1566. doi: 10.1039/d2ra06101e
Giuliani C. (2019). The flavonoid quercetin induces AP-1 activation in FRTL-5 thyroid cells. Antioxidants (Basel) 8 (5), 112. doi: 10.3390/antiox8050112
Guo X., Wang Y., Qin Y., Shen P., Peng Q. (2020). Structures, properties and application of alginic acid: A review. Int. J. Biol. Macromol 162, 618–628. doi: 10.1016/j.ijbiomac.2020.06.180
Ha H. T., Cuong D. X., Thuy L. H., Thuan P. T., Tuyen D. T. T., Mo V. T., et al. (2022). Carrageenan of red algae eucheuma gelatinae: extraction, antioxidant activity, rheology characteristics, and physicochemistry characterization. Molecules 27 (4), 1268. doi: 10.3390/molecules27041268
Hakimi F., Jafari H., Hashemikia S., Shabani S., Ramazani A. (2023). Chitosan-polyethylene oxide/clay-alginate nanofiber hydrogel scaffold for bone tissue engineering: Preparation, physical characterization, and biomimetic mineralization. Int. J. Biol. Macromol 233, 123453. doi: 10.1016/j.ijbiomac.2023.123453
Harada N., Maeda M. (1998). Chemical structure of antithrombin-active Rhamnan sulfate from Monostrom nitidum. Biosci. Biotechnol. Biochem. 62 (9), 1647–1652. doi: 10.1271/bbb.62.1647
Healy L. E., Zhu X., Pojic M., Sullivan C., Tiwari U., Curtin J., et al. (2023). Biomolecules from macroalgae-nutritional profile and bioactives for novel food product development. Biomolecules 13 (2), 386. doi: 10.3390/biom13020386
Hoseinpour V., Shariatinia Z. (2021). Applications of zeolitic imidazolate framework-8 (ZIF-8) in bone tissue engineering: A review. Tissue Cell 72, 101588. doi: 10.1016/j.tice.2021.101588
Hsu F. Y., Chen J. J., Sung W. C., Hwang P. A. (2021). Preparation of a fucoidan-grafted hyaluronan composite hydrogel for the induction of osteoblast differentiation in osteoblast-like cells. Materials (Basel) 14 (5), 1168. doi: 10.3390/ma14051168
Huang X., Zhang X., Wang X., Wang C., Tang B. (2012). Microenvironment of alginate-based microcapsules for cell culture and tissue engineering. J. Biosci. Bioeng 114 (1), 1–8. doi: 10.1016/j.jbiosc.2012.02.024
Huang Q., Zou Y., Arno M. C., Chen S., Wang T., Gao J., et al. (2017). Hydrogel scaffolds for differentiation of adipose-derived stem cells. Chem. Soc. Rev. 46 (20), 6255–6275. doi: 10.1039/c6cs00052e
Ibrahim M. I. A., Amer M. S., Ibrahim H. A. H., Zaghloul E. H. (2022). Considerable production of ulvan from ulva lactuca with special emphasis on its antimicrobial and anti-fouling properties. Appl. Biochem. Biotechnol. 194 (7), 3097–3118. doi: 10.1007/s12010-022-03867-y
Ingavle G. C., Gionet-Gonzales M., Vorwald C. E., Bohannon L. K., Clark K., Galuppo L. D., et al. (2019). Injectable mineralized microsphere-loaded composite hydrogels for bone repair in a sheep bone defect model. Biomaterials 197, 119–128. doi: 10.1016/j.biomaterials.2019.01.005
Jain A., Bansal K. K., Tiwari A., Rosling A., Rosenholm J. M. (2018). Role of polymers in 3D printing technology for drug delivery - an overview. Curr. Pharm. Des. 24 (42), 4979–4990. doi: 10.2174/1381612825666181226160040
Jayawardena T. U., Nagahawatta D. P., Fernando I. P. S., Kim Y. T., Kim J. S., Kim W. S., et al. (2022). A review on fucoidan structure, extraction techniques, and its role as an immunomodulatory agent. Mar. Drugs 20 (12), 755. doi: 10.3390/md20120755
Jiang P., Yan C., Guo Y., Zhang X., Cai M., Jia X., et al. (2019). Direct ink writing with high-strength and swelling-resistant biocompatible physically crosslinked hydrogels. Biomater Sci. 7 (5), 1805–1814. doi: 10.1039/c9bm00081j
Kikionis S., Ioannou E., Aggelidou E., Tziveleka L. A., Demiri E., Bakopoulou A., et al. (2021). The marine polysaccharide ulvan confers potent osteoinductive capacity to PCL-based scaffolds for bone tissue engineering applications. Int. J. Mol. Sci. 22 (6), 3086. doi: 10.3390/ijms22063086
Kikionis S., Koromvoki M., Tagka A., Polichronaki E., Stratigos A., Panagiotopoulos A., et al. (2022). Ulvan-based nanofibrous patches enhance wound healing of skin trauma resulting from cryosurgical treatment of keloids. Mar. Drugs 20 (9), 551. doi: 10.3390/md20090551
Kim B. S., Yang S. S., You H. K., Shin H. I., Lee J. (2018). Fucoidan-induced osteogenic differentiation promotes angiogenesis by inducing vascular endothelial growth factor secretion and accelerates bone repair. J. Tissue Eng. Regener. Med. 12 (3), e1311–e1324. doi: 10.1002/term.2509
Kothale D., Verma U., Dewangan N., Jana P., Jain A., Jain D. (2020). Alginate as promising natural polymer for pharmaceutical, food, and biomedical applications. Curr. Drug Delivery 17 (9), 755–775. doi: 10.2174/1567201817666200810110226
Kumari S., Mondal P., Chatterjee K. (2022). Digital light processing-based 3D bioprinting of kappa-carrageenan hydrogels for engineering cell-loaded tissue scaffolds. Carbohydr Polym 290, 119508. doi: 10.1016/j.carbpol.2022.119508
Kwack K. H., Ji J. Y., Park B., Heo J. S. (2022). Fucoidan (Undaria pinnatifida)/polydopamine composite-modified surface promotes osteogenic potential of periodontal ligament stem cells. Mar. Drugs 20 (3), 181. doi: 10.3390/md20030181
Labowska M. B., Skrodzka M., Sicinska H., Michalak I., Detyna J. (2023). Influence of cross-linking conditions on drying kinetics of alginate hydrogel. Gels 9 (1), 63. doi: 10.3390/gels9010063
Lakshmi D. S., Sankaranarayanan S., Gajaria T. K., Li G., Kujawski W., Kujawa J., et al. (2020). A short review on the valorization of green seaweeds and ulvan: FEEDSTOCK for chemicals and biomaterials. Biomolecules 10 (7), 991. doi: 10.3390/biom10070991
Lee J., Byun H., Madhurakkat Perikamana S. K., Lee S., Shin H. (2019). Current advances in immunomodulatory biomaterials for bone regeneration. Adv. Healthc Mater 8 (4), e1801106. doi: 10.1002/adhm.201801106
Lertwimol T., Sonthithai P., Hankamolsiri W., Kaewkong P., Uppanan P. (2022). Development of chondrocyte-laden alginate hydrogels with modulated microstructure and properties for cartilage regeneration. Biotechnol. Prog. 39 (2), e3322. doi: 10.1002/btpr.3322
Li Z., Chen X., Bao C., Liu C., Liu C., Li D., et al. (2021). Fabrication and evaluation of alginate/bacterial cellulose nanocrystals-chitosan-gelatin composite scaffolds. Molecules 26 (16), 5003. doi: 10.3390/molecules26165003
Li B., Lu F., Wei X., Zhao R. (2008). Fucoidan: structure and bioactivity. Molecules 13 (8), 1671–1695. doi: 10.3390/molecules13081671
Li Y., McGowan E., Chen S., Santos J., Yin H., Lin Y. (2023a). Immunopotentiating activity of fucoidans and relevance to cancer immunotherapy. Mar. Drugs 21 (2), 128. doi: 10.3390/md21020128
Li L., Ni R., Shao Y., Mao S. (2014). Carrageenan and its applications in drug delivery. Carbohydr Polym 103, 1–11. doi: 10.1016/j.carbpol.2013.12.008
Li Y., Ren X., Zhu L., Li C. (2023b). Biomass 3D printing: principles, materials, post-processing and applications. Polymers (Basel) 15 (12), 2692. doi: 10.3390/polym15122692
Li Y., Xu Z., Wang J., Pei X., Chen J., Wan Q. (2023c). Alginate-based biomaterial-mediated regulation of macrophages in bone tissue engineering. Int. J. Biol. Macromol 230, 123246. doi: 10.1016/j.ijbiomac.2023.123246
Lim W., Kim G. J., Kim H. W., Lee J., Zhang X., Kang M. G., et al. (2020). Kappa-carrageenan-based dual crosslinkable bioink for extrusion type bioprinting. Polymers (Basel) 12 (10), 2377. doi: 10.3390/polym12102377
Liu Y., Chan J. K., Teoh S. H. (2015). Review of vascularised bone tissue-engineering strategies with a focus on co-culture systems. J. Tissue Eng. Regener. Med. 9 (2), 85–105. doi: 10.1002/term.1617
Liu F., Duan G., Yang H. (2023). Recent advances in exploiting carrageenans as a versatile functional material for promising biomedical applications. Int. J. Biol. Macromol 235, 123787. doi: 10.1016/j.ijbiomac.2023.123787
Liu Y., Hu Q., Dong W., Liu S., Zhang H., Gu Y. (2022). Alginate/gelatin-based hydrogel with soy protein/peptide powder for 3D printing tissue-engineering scaffolds to promote angiogenesis. Macromol Biosci. 22 (4), e2100413. doi: 10.1002/mabi.202100413
Liu D., Ouyang Y., Chen R., Wang M., Ai C., El-Seedi H. R., et al. (2022). Nutraceutical potentials of seaweed ulvan for healthy aging. Int. J. Biol. Macromol 194, 422–434. doi: 10.1016/j.ijbiomac.2021.11.084
Lomartire S., Goncalves A. M. M. (2022). An overview of potential seaweed-derived bioactive compounds for pharmaceutical applications. Mar. Drugs 20 (2), 141. doi: 10.3390/md20020141
Lopes D., Martins-Cruz C., Oliveira M. B., Mano J. F. (2018). Bone physiology as inspiration for tissue regenerative therapies. Biomaterials 185, 240–275. doi: 10.1016/j.biomaterials.2018.09.028
Loukelis K., Papadogianni D., Chatzinikolaidou M. (2022). Kappa-carrageenan/chitosan/gelatin scaffolds enriched with potassium chloride for bone tissue engineering. Int. J. Biol. Macromol 209 (Pt B), 1720–1730. doi: 10.1016/j.ijbiomac.2022.04.129
Lu H. T., Lu T. W., Chen C. H., Mi F. L. (2019). Development of genipin-crosslinked and fucoidan-adsorbed nano-hydroxyapatite/hydroxypropyl chitosan composite scaffolds for bone tissue engineering. Int. J. Biol. Macromol 128, 973–984. doi: 10.1016/j.ijbiomac.2019.02.010
Mabate B., Daub C. D., Malgas S., Edkins A. L., Pletschke B. I. (2021). Fucoidan structure and its impact on glucose metabolism: implications for diabetes and cancer therapy. Mar. Drugs 19 (1), 30. doi: 10.3390/md19010030
Mahendiran B., Muthusamy S., Sampath S., Jaisankar S. N., Popat K. C., Selvakumar R., et al. (2021). Recent trends in natural polysaccharide based bioinks for multiscale 3D printing in tissue regeneration: A review. Int. J. Biol. Macromol 183, 564–588. doi: 10.1016/j.ijbiomac.2021.04.179
Mandal S., Nagi G. K., Corcoran A. A., Agrawal R., Dubey M., Hunt R. W. (2023). Seaweed polysaccharides for 3D printing: A review. Carbohydr Polym 300, 120267. doi: 10.1016/j.carbpol.2022.120267
Manlusoc J. K. T., Hsieh C. L., Hsieh C. Y., Salac E. S. N., Lee Y. T., Tsai P. W. (2019). Pharmacologic application potentials of sulfated polysaccharide from marine algae. Polymers (Basel) 11 (7), 1163. doi: 10.3390/polym11071163
McKinley T. O., Natoli R. N., Janakiram N. B., Warden S. J., Fuchs R. K., Gunderson Z., et al. (2023). Minced Muscle Autografting Improves Bone Healing but not Muscle Function in a Porcine Composite Injury Model. J. Orthop Res. 41 (9), 1890–1901. doi: 10.1002/jor.25551
Moncada D., Rico M., Montero B., Rodriguez-Llamazares S., Feijoo-Bandin S., Gualillo O., et al. (2023). Injectable hybrid hydrogels physically crosslinked based on carrageenan and green graphene for tissue repair. Int. J. Biol. Macromol 235, 123777. doi: 10.1016/j.ijbiomac.2023.123777
Muscolino E., Costa M. A., Sabatino M. A., Alessi S., Bulone D., San Biagio P. L., et al. (2022a). Recombinant mussel protein Pvfp5beta enhances cell adhesion of poly(vinyl alcohol)/k-carrageenan hydrogel scaffolds. Int. J. Biol. Macromol 211, 639–652. doi: 10.1016/j.ijbiomac.2022.05.068
Muscolino E., Di Stefano A. B., Trapani M., Sabatino M. A., Giacomazza D., Alessi S., et al. (2022b). kappa-Carrageenan and PVA blends as bioinks to 3D print scaffolds for cartilage reconstruction. Int. J. Biol. Macromol 222 (Pt B), 1861–1875. doi: 10.1016/j.ijbiomac.2022.09.275
Newman H., Shih Y. V., Varghese S. (2021). Resolution of inflammation in bone regeneration: From understandings to therapeutic applications. Biomaterials 277, 121114. doi: 10.1016/j.biomaterials.2021.121114
Ngo D. H., Kim S. K. (2013). Sulfated polysaccharides as bioactive agents from marine algae. Int. J. Biol. Macromol 62, 70–75. doi: 10.1016/j.ijbiomac.2013.08.036
Nielsen M. S., Mikkelsen M. D., Ptak S. H., Hejbol E. K., Ohmes J., Thi T. N., et al. (2022). Efficacy of marine bioactive compound fucoidan for bone regeneration and implant fixation in sheep. J. BioMed. Mater Res. A 110 (4), 861–872. doi: 10.1002/jbm.a.37334
Nourmohammadi J., Roshanfar F., Farokhi M., Haghbin Nazarpak M. (2017). Silk fibroin/kappa-carrageenan composite scaffolds with enhanced biomimetic mineralization for bone regeneration applications. Mater Sci. Eng. C Mater Biol. Appl. 76, 951–958. doi: 10.1016/j.msec.2017.03.166
Nunes C., Coimbra M. A. (2019). The potential of fucose-containing sulfated polysaccharides as scaffolds for biomedical applications. Curr. Med. Chem. 26 (35), 6399–6411. doi: 10.2174/0929867326666181213093718
Ohmes J., Mikkelsen M. D., Nguyen T. T., Tran V. H. N., Meier S., Nielsen M. S., et al. (2022). Depolymerization of fucoidan with endo-fucoidanase changes bioactivity in processes relevant for bone regeneration. Carbohydr Polym 286, 119286. doi: 10.1016/j.carbpol.2022.119286
Oliyaei N., Moosavi-Nasab M., Mazloomi S. M. (2022). Therapeutic activity of fucoidan and carrageenan as marine seaweed polysaccharides against viruses. 3 Biotech. 12 (7), 154. doi: 10.1007/s13205-022-03210-6
Pajovich H. T., Banerjee I. A. (2017). Biomineralization of fucoidan-peptide blends and their potential applications in bone tissue regeneration. J. Funct. Biomater 8 (3), 41. doi: 10.3390/jfb8030041
Papagiannopoulos A., Nikolakis S. P., Pamvouxoglou A., Koutsopoulou E. (2023). Physicochemical properties of electrostatically crosslinked carrageenan/chitosan hydrogels and carrageenan/chitosan/Laponite nanocomposite hydrogels. Int. J. Biol. Macromol 225, 565–573. doi: 10.1016/j.ijbiomac.2022.11.113
Popa E. G., Reis R. L., Gomes M. E. (2015). Seaweed polysaccharide-based hydrogels used for the regeneration of articular cartilage. Crit. Rev. Biotechnol. 35 (3), 410–424. doi: 10.3109/07388551.2014.889079
Prajapati V. D., Maheriya P. M., Jani G. K., Solanki H. K. (2014). Carrageenan: a natural seaweed polysaccharide and its applications. Carbohydr Polym 105, 97–112. doi: 10.1016/j.carbpol.2014.01.067
Purnama A., Aid-Launais R., Haddad O., Maire M., Mantovani D., Letourneur D., et al. (2015). Fucoidan in a 3D scaffold interacts with vascular endothelial growth factor and promotes neovascularization in mice. Drug Delivery Transl. Res. 5 (2), 187–197. doi: 10.1007/s13346-013-0177-4
Qin X., Pan H., Yang K., Xie W., Yang G., Wang J. (2023). Biodegradable and biocompatible alginate/gelatin/MXene composite membrane with efficient osteogenic activity and its application in guided bone regeneration. J. Biomater Sci. Polym Ed 34 (13), 1843–1857. doi: 10.1080/09205063.2023.2187987
Quinlan E., Lopez-Noriega A., Thompson E. M., Hibbitts A., Cryan S. A., O'Brien F. J. (2017). Controlled release of vascular endothelial growth factor from spray-dried alginate microparticles in collagen-hydroxyapatite scaffolds for promoting vascularization and bone repair. J. Tissue Eng. Regener. Med. 11 (4), 1097–1109. doi: 10.1002/term.2013
Qureshi D., Nayak S. K., Maji S., Kim D., Banerjee I., Pal K. (2019). Carrageenan: A wonder polymer from marine algae for potential drug delivery applications. Curr. Pharm. Des. 25 (11), 1172–1186. doi: 10.2174/1381612825666190425190754
Ren C. G., Liu Z. Y., Wang X. L., Qin S. (2022). The seaweed holobiont: from microecology to biotechnological applications. Microb. Biotechnol. 15 (3), 738–754. doi: 10.1111/1751-7915.14014
Resmi R., Parvathy J., John A., Joseph R. (2020). Injectable self-crosslinking hydrogels for meniscal repair: A study with oxidized alginate and gelatin. Carbohydr Polym 234, 115902. doi: 10.1016/j.carbpol.2020.115902
Roshanfar F., Hesaraki S., Dolatshahi-Pirouz A. (2022). Electrospun silk fibroin/kappa-carrageenan hybrid nanofibers with enhanced osteogenic properties for bone regeneration applications. Biol. (Basel) 11 (5), 751. doi: 10.3390/biology11050751
Sadowska J. M., Ginebra M. P. (2020). Inflammation and biomaterials: role of the immune response in bone regeneration by inorganic scaffolds. J. Mater Chem. B 8 (41), 9404–9427. doi: 10.1039/d0tb01379j
Sairaman S., Nivedhitha M. S., Shrivastava D., Al Onazi M. A., Algarni H. A., Mustafa M., et al. (2022). Biocompatibility and antioxidant activity of a novel carrageenan based injectable hydrogel scaffold incorporated with Cissus quadrangularis: an in vitro study. BMC Oral. Health 22 (1), 377. doi: 10.1186/s12903-022-02409-6
Saravana P. S., Cho Y. N., Patil M. P., Cho Y. J., Kim G. D., Park Y. B., et al. (2018). Hydrothermal degradation of seaweed polysaccharide: Characterization and biological activities. Food Chem. 268, 179–187. doi: 10.1016/j.foodchem.2018.06.077
Schlundt C., Fischer H., Bucher C. H., Rendenbach C., Duda G. N., Schmidt-Bleek K. (2021). The multifaceted roles of macrophages in bone regeneration: A story of polarization, activation and time. Acta Biomater 133, 46–57. doi: 10.1016/j.actbio.2021.04.052
Seedevi P., Moovendhan M., Viramani S., Shanmugam A. (2017). Bioactive potential and structural chracterization of sulfated polysaccharide from seaweed (Gracilaria corticata). Carbohydr Polym 155, 516–524. doi: 10.1016/j.carbpol.2016.09.011
Sharma A., Kaur I., Dheer D., Nagpal M., Kumar P., Venkatesh D. N., et al. (2023). A propitious role of marine sourced polysaccharides: Drug delivery and biomedical applications. Carbohydr Polym 308, 120448. doi: 10.1016/j.carbpol.2022.120448
Sikkema R., Keohan B., Zhitomirsky I. (2021). Alginic acid polymer-hydroxyapatite composites for bone tissue engineering. Polymers (Basel) 13 (18), 3070. doi: 10.3390/polym13183070
Sulastri E., Zubair M. S., Lesmana R., Mohammed A. F. A., Wathoni N. (2021). Development and characterization of ulvan polysaccharides-based hydrogel films for potential wound dressing applications. Drug Des. Devel Ther. 15, 4213–4226. doi: 10.2147/DDDT.S331120
Taghipour Y. D., Hokmabad V. R., Del Bakhshayesh A. R., Asadi N., Salehi R., Nasrabadi H. T. (2020). The application of hydrogels based on natural polymers for tissue engineering. Curr. Med. Chem. 27 (16), 2658–2680. doi: 10.2174/0929867326666190711103956
Tanaka R., Kurishiba Y., Miyake H., Shibata T. (2022). Isolation, diversity and characterization of ulvan-degrading bacteria isolated from marine environments. Molecules 27 (11), 3420. doi: 10.3390/molecules27113420
Tavakoli S., Kharaziha M., Kermanpur A., Mokhtari H. (2019). Sprayable and injectable visible-light Kappa-carrageenan hydrogel for in-situ soft tissue engineering. Int. J. Biol. Macromol 138, 590–601. doi: 10.1016/j.ijbiomac.2019.07.126
Thye K. L., Wan Abdullah W., Balia Yusof Z. N., Wee C. Y., Ong-Abdullah J., Loh J. Y., et al. (2022). lambda-Carrageenan promotes plant growth in banana via enhancement of cellular metabolism, nutrient uptake, and cellular homeostasis. Sci. Rep. 12 (1), 19639. doi: 10.1038/s41598-022-21909-7
Tohamy K. M., Soliman I. E., Mabrouk M., ElShebiney S., Beherei H. H., Aboelnasr M. A., et al. (2018). Novel polysaccharide hybrid scaffold loaded with hydroxyapatite: Fabrication, bioactivity, and in vivo study. Mater Sci. Eng. C Mater Biol. Appl. 93, 1–11. doi: 10.1016/j.msec.2018.07.054
Tran P. H. L., Lee B. J., Tran T. T. D. (2021). Current developments in the oral drug delivery of fucoidan. Int. J. Pharm. 598, 120371. doi: 10.1016/j.ijpharm.2021.120371
Tziveleka L. A., Ioannou E., Roussis V. (2019). Ulvan, a bioactive marine sulphated polysaccharide as a key constituent of hybrid biomaterials: A review. Carbohydr Polym 218, 355–370. doi: 10.1016/j.carbpol.2019.04.074
Tziveleka L. A., Sapalidis A., Kikionis S., Aggelidou E., Demiri E., Kritis A., et al. (2020). Hybrid sponge-like scaffolds based on ulvan and gelatin: design, characterization and evaluation of their potential use in bone tissue engineering. Materials (Basel) 13 (7), 1763. doi: 10.3390/ma13071763
Usov A. I. (2011). Polysaccharides of the red algae. Adv. Carbohydr Chem. Biochem. 65, 115–217. doi: 10.1016/B978-0-12-385520-6.00004-2
Valcarcel J., Novoa-Carballal R., Perez-Martin R. I., Reis R. L., Vazquez J. A. (2017). Glycosaminoglycans from marine sources as therapeutic agents. Biotechnol. Adv. 35 (6), 711–725. doi: 10.1016/j.bioteChadv.2017.07.008
van Weelden G., Bobinski M., Okla K., van Weelden W. J., Romano A., Pijnenborg J. M. A. (2019). Fucoidan structure and activity in relation to anti-cancer mechanisms. Mar. Drugs 17 (1), 32. doi: 10.3390/md17010032
Venkatesan J., Anil S., Rao S., Bhatnagar I., Kim S. K. (2019). Sulfated polysaccharides from macroalgae for bone tissue regeneration. Curr. Pharm. Des. 25 (11), 1200–1209. doi: 10.2174/1381612825666190425161630
Venkatesan J., Bhatnagar I., Manivasagan P., Kang K. H., Kim S. K. (2015). Alginate composites for bone tissue engineering: a review. Int. J. Biol. Macromol 72, 269–281. doi: 10.1016/j.ijbiomac.2014.07.008
Veronesi F., Maglio M., Brogini S., Fini M. (2020). In vivo studies on osteoinduction: A systematic review on animal models, implant site, and type and postimplantation investigation. J. BioMed. Mater Res. A 108 (9), 1834–1866. doi: 10.1002/jbm.a.36949
Walsh P. J., McGrath S., McKelvey S., Ford L., Sheldrake G., Clarke S. A. (2019). The osteogenic potential of brown seaweed extracts. Mar. Drugs 17 (3), 141. doi: 10.3390/md17030141
Wang X., Guo C., Pi M., Li M., Yang X., Lu H., et al. (2022). Significant roles of ions in enhancing and functionalizing anisotropic hydrogels. ACS Appl. Mater Interfaces 14 (45), 51318–51328. doi: 10.1021/acsami.2c15138
Wang N., Tian J., Wang L., Song S., Ai C., Janaswamy S., et al. (2021). Fucoidan hydrogels induced by kappa-carrageenan: Rheological, thermal and structural characterization. Int. J. Biol. Macromol 191, 514–520. doi: 10.1016/j.ijbiomac.2021.09.111
Wu T., Liu L., Gao Z., Cui C., Fan C., Liu Y., et al. (2023). Extracellular matrix (ECM)-inspired high-strength gelatin-alginate based hydrogels for bone repair. Biomater Sci. 11 (8), 2877–2885. doi: 10.1039/d3bm00213f
Xu S. Y., Huang X., Cheong K. L. (2017). Recent advances in marine algae polysaccharides: isolation, structure, and activities. Mar. Drugs 15 (12), 388. doi: 10.3390/md15120388
Yadav D., Song M. (2022). Therapeutic applications of fucoidans and their potential to act against COVID-19. Curr. Pharm. Des. 28 (46), 3671–3676. doi: 10.2174/1381612829666221207093215
Yegappan R., Selvaprithiviraj V., Amirthalingam S., Jayakumar R. (2018). Carrageenan based hydrogels for drug delivery, tissue engineering and wound healing. Carbohydr Polym 198, 385–400. doi: 10.1016/j.carbpol.2018.06.086
Yu Q., Han F., Yuan Z., Zhu Z., Liu C., Tu Z., et al. (2022). Fucoidan-loaded nanofibrous scaffolds promote annulus fibrosus repair by ameliorating the inflammatory and oxidative microenvironments in degenerative intervertebral discs. Acta Biomater 148, 73–89. doi: 10.1016/j.actbio.2022.05.054
Zayed A., El-Aasr M., Ibrahim A. S., Ulber R. (2020). Fucoidan characterization: determination of purity and physicochemical and chemical properties. Mar. Drugs 18 (11), 571. doi: 10.3390/md18110571
Zhang L., Yang G., Johnson B. N., Jia X. (2019). Three-dimensional (3D) printed scaffold and material selection for bone repair. Acta Biomater 84, 16–33. doi: 10.1016/j.actbio.2018.11.039
Zhao D., Wang X., Cheng B., Yin M., Hou Z., Li X., et al. (2022). Degradation-kinetics-controllable and tissue-regeneration-matchable photocross-linked alginate hydrogels for bone repair. ACS Appl. Mater Interfaces 14 (19), 21886–21905. doi: 10.1021/acsami.2c01739
Zheng W., Hao Y., Wang D., Huang H., Guo F., Sun Z., et al. (2021). Preparation of triamcinolone acetonide-loaded chitosan/fucoidan hydrogel and its potential application as an oral mucosa patch. Carbohydr Polym 272, 118493. doi: 10.1016/j.carbpol.2021.118493
Keywords: seaweed polysaccharide, polysaccharide sulfate, bone regeneration, novel formulation, regenerative medicine
Citation: Jin Y, Yu Q, Li S, Chen T and Liu D (2023) Application of seaweed polysaccharide in bone tissue regeneration. Front. Mar. Sci. 10:1202422. doi: 10.3389/fmars.2023.1202422
Received: 08 April 2023; Accepted: 08 September 2023;
Published: 26 September 2023.
Edited by:
Suresh Veeraperumal, Upstate Medical University, United StatesReviewed by:
Esra Imamoglu, Ege University, TürkiyeLeonel Pereira, University of Coimbra, Portugal
Chetan Paliwal, Centrum Algatech, Czechia
Weiqi Fu, Zhejiang University, China
Copyright © 2023 Jin, Yu, Li, Chen and Liu. This is an open-access article distributed under the terms of the Creative Commons Attribution License (CC BY). The use, distribution or reproduction in other forums is permitted, provided the original author(s) and the copyright owner(s) are credited and that the original publication in this journal is cited, in accordance with accepted academic practice. No use, distribution or reproduction is permitted which does not comply with these terms.
*Correspondence: Da Liu, liuda_1986@163.com; Tianli Chen, tli_chen@163.com
†These authors have contributed equally to this work